Ammonia Cracking
Ammonia (NH3) is a vital molecule in the chemical industry, and its large-scale production is essential for manufacturing nitrogen fertilisers, industrial chemicals and a variety of other compounds. The ammonia cracking process, also known as thermal decomposition of ammonia, plays a key role within the production of molecular nitrogen (N2) and hydrogen (H2) from ammonia. This chemical process is essential and has a major impact on the production of fertilisers, industrial chemicals and fuels, since it involves the thermal decomposition of ammonia (NH3) into its components, nitrogen (N2) and hydrogen (H2), at very high temperatures. Ammonia cracking also has crucial industrial applications, particularly in the production of hydrogen, an essential gas in the chemical and energy industries. This article will explore this chemical process in detail, covering its foundations, its various applications, its relevance in the industry and the future challenges to be faced.

Introduction to ammonia cracking
Ammonia is a molecule composed of one nitrogen atom (N) and three hydrogen atoms (H). Its chemical formula is NH3, and it is a gaseous molecule at ambient temperature and pressure. Ammonia is commercially obtained by the Haber-Bosch synthesis, which combines atmospheric nitrogen with molecular hydrogen to form NH3. This reaction is expressed as follows:
N2 + 3H2 ⇌ 2NH3
The importance of ammonia cracking lies in its ability to generate two high-value chemicals: hydrogen and nitrogen.
- Hydrogen production: Hydrogen is an essential element in many industrial processes and it is key to the production of a variety of products, including synthetic ammonia, fine chemicals and clean fuels. Hydrogen is also considered a promising energy source in applications such as fuel cells and energy storage.
- Nitrogen production: Nitrogen is also crucial in the chemical industry, notably in the manufacture of fertilisers and nitrogen chemicals. It is also used in applications such as inertisation in the chemical process industry and food preservation.
The efficient production of hydrogen and nitrogen by cracking ammonia plays an essential role in meeting the growing demand for these essential chemicals as well as in reducing the dependence on non-renewable energy sources for hydrogen generation. Furthermore, this process can have a significant impact towards reducing carbon emissions when combined with carbon capture and storage technologies.
Process description and thermodynamics
Ammonia cracking is a chemical process that involves the thermal decomposition of ammonia into its main components, molecular nitrogen (N2) and molecular hydrogen (H2). This process is carried out at high temperatures and pressures and it is used to obtain pure hydrogen, which is a high-value product in the chemical industry. The general chemical equation for cracking ammonia is expressed as follows:
2NH3 ⇌ N2 + 3H2
However, the aforementioned simplification does not reflect the real complexity that arises during the intermediate stages of the process, neither considers the possible production of by-products. Thermodynamics plays a key role in the investigation of the energy and balance conditions that regulate these reactions, which in turn leads to the determination of the optimal conditions for producing hydrogen and nitrogen in their purest state.
The ammonia cracking reaction is endothermic, which means that it requires a substantial amount of energy to break the chemical bonds within ammonia. Elevated temperatures (usually between 800 and 1000 degrees Celsius) and high pressures are required for ammonia to be decomposed into nitrogen and hydrogen, as indicated in the above-mentioned equation. The process involves the splitting of the N-H bonds in ammonia, followed by the formation of N-N and H-H bonds respectively for nitrogen and hydrogen.
It must be highlighted that this process involves significant energy consumption. Reactors specifically designed to withstand high temperature and pressure conditions are used to carry out ammonia cracking. Whilst operating conditions may vary according to the design and capacity of the reactor, they are usually held in a temperature range between 800 and 1000 degrees Celsius and a pressure ranging between 50 and 200 atmospheres. These extreme conditions are vital to achieve an acceptable reaction rate and maximise the conversion of ammonia into nitrogen and hydrogen.
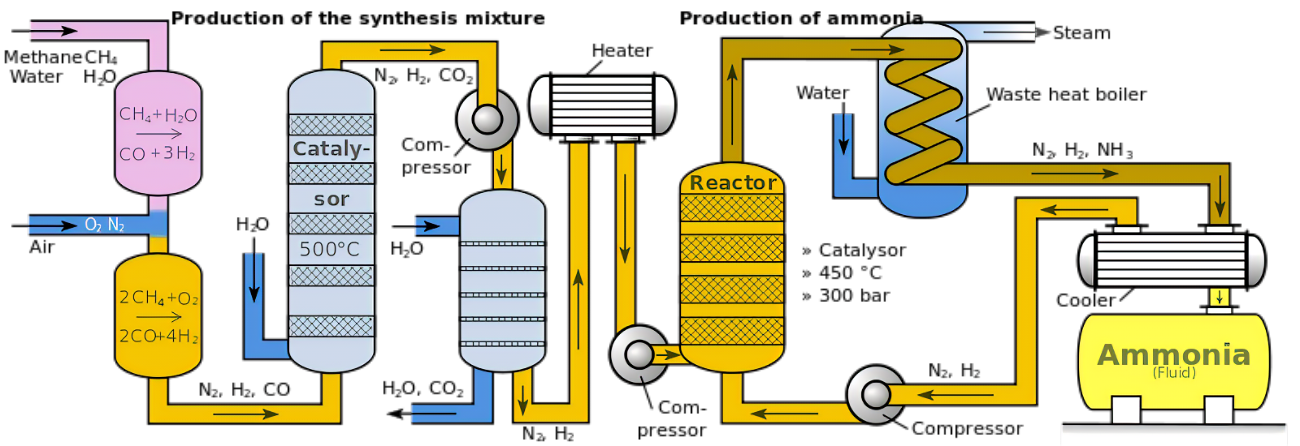
Ammonia cracking kinetics is concerned with understanding how the reaction rate varies as a response to different conditions, such as temperature, pressure and the presence of catalysts. Temperature is the dominant factor influencing the rate of this reaction, increasing significantly as the temperature rises. However, this temperature rise can also increase the generation of undesirable by-products, which requires a precise control of the temperature to obtain the purest product.
Pressure is another crucial factor affecting kinetics. An increase in pressure can favour hydrogen generation, although it must be carefully balanced with safety and security considerations. Moreover, the choice and presence of catalysts can accelerate the reaction, improving the selectivity and efficiency of hydrogen and nitrogen production.
Besides these factors, kinetics is also influenced by the ratio of reactant to product concentration, as well as by the characteristics of the reactor used, including its design and its size.
To sum up, understanding the thermodynamics and kinetics of ammonia cracking is essential to optimise this chemical process, thereby allowing the efficient production of hydrogen and nitrogen, whilst minimising undesirable by-products.
Catalysts
A catalyst is often used to increase the efficiency of the ammonia cracking process. Catalysts are substances that accelerate chemical reactions without being consumed in the reaction itself. When cracking ammonia, catalysts can improve the rate of ammonia decomposition and reduce the temperature and pressure required for this process.
- Types of catalysts: Typical catalysts include noble metals such as platinum (Pt), ruthenium (Ru) and palladium (Pd), as well as metal oxides such as iron oxide (Fe2O3) and aluminium oxide (Al2O3). Each of these catalysts has specific properties that influence the decomposition of ammonia and the formation of hydrogen and nitrogen.
- Efficiency effects: Catalysts can significantly increase the efficiency of the ammonia cracking process by reducing the temperature required for the reaction. Apart from decreasing energy consumption, this can also extend the lifetime of the equipment and decrease production costs. Furthermore, some catalysts can promote the complete conversion of ammonia to hydrogen and nitrogen, minimising the formation of undesirable by-products.
- Effects on selectivity: The choice of catalyst can influence the selectivity of the products obtained. Some catalysts may favour the production of hydrogen over nitrogen, while others may enhance the selectivity towards nitrogen. This is especially relevant in specific applications where pure chemicals are required. Optimising selectivity is essential for industrial production to ensure the quality of the final products.
- Challenges and considerations: While catalysts can significantly improve the ammonia cracking process, they can also present challenges. As an example, some catalysts may suffer from poisoning or deterioration over time due to the accumulation of impurities or adverse operating conditions. Therefore, proper catalyst selection and maintenance are both critical to ensure the ongoing efficiency of the reaction.
Finally, the research and development of efficient and selective catalysts in ammonia cracking are active areas of study in chemistry and process engineering. The aim is to find catalysts that allow efficient and sustainable production of hydrogen and nitrogen, thus supporting the chemical and energy industry’s progress towards a cleaner and more efficient future.
Ammonia cracking processes and technologies
Some common types of ammonia cracking are presented below:
- Thermal cracking of ammonia: This is the simplest type of ammonia cracking and is performed at high temperatures (around 1000 degrees Celsius) without the presence of a catalyst. Ammonia is decomposed into nitrogen and hydrogen due to heat. This process is endothermic, which means that a large amount of energy is required as heat.
- Catalytic cracking of ammonia: Here, a catalyst is used to reduce the temperature required for the decomposition of ammonia. A commonly used catalyst is platinum (Pt), which accelerates the decomposition reaction to lower temperatures, saving energy and reducing costs.
- Steam cracking of ammonia: In this process, ammonia is combined with steam (H2O) and subjected to elevated temperatures in the presence of a catalyst. The steam then reacts with the ammonia to produce nitrogen and hydrogen, which can be beneficial in terms of thermodynamic equilibrium and efficiency.
Industrial applications of ammonia cracking
Hydrogen and nitrogen obtained through the ammonia cracking process have a wide range of industrial applications across several industries, playing a crucial role in chemistry, petrochemicals, energy and even more. Some of the most noteworthy applications are examined below:
- Petrochemical industry: hydrogen and nitrogen are essential components in the petrochemical industry. Hydrogen is used in the hydro desulphurisation (removal of sulphur) and hydrogenation (addition of hydrogen) of organic compounds, key processes in the production of clean fuels and fine chemicals. Nitrogen is used in the inerting of equipment and storage of chemicals to prevent oxidation and explosion.
- Energy and Fuel Cells: Hydrogen produced from cracking ammonia has great potential in clean energy production. It can be used in fuel cells to produce electricity with zero emissions of polluting gases, thus being a promising alternative for sustainable mobility and stationary power production.
- Synthetic Ammonia Production: Hydrogen and nitrogen are the main ingredients in the production of synthetic ammonia (NH3), which is essential for the manufacture of fertilisers. Modern agriculture relies heavily on nitrogen fertilisers, making ammonia a high-value chemical.
- Production of Fine Chemicals: Both hydrogen and nitrogen are used in the synthesis of a wide variety of fine chemicals and advanced materials. This includes the manufacture of pharmaceuticals, high-performance chemicals and high-tech materials.
- Electronics and Metallurgy: Nitrogen is used in the electronics industry to create controlled atmospheres in the production of semiconductors and electronic devices. It is also used in metallurgy to improve the quality of metals and reduce oxidation.
- Energy Storage and Cryogenic Refrigeration: Hydrogen can be used as an energy storage medium, since it can be converted back into electricity when required. Liquid nitrogen is used in cryogenic refrigeration applications, such as the preservation of biological tissues and the production of superconducting materials.
To summarise, hydrogen and nitrogen produced by ammonia cracking are essential resources in several industries, playing a key role in the production of clean energy, essential chemical products and advanced materials. Its versatility and diversified applications make the ammonia cracking process an integral part of modern industrial infrastructure and represent an important contribution to sustainability and efficiency in the industrial production.

Ammonia as an energy carrier
Ammonia could play a key role within the transition to renewable fuels. It is a high-quality energy carrier, particularly as a hydrogen storage vehicle.
The development of the energy transition is a major challenge for all sectors of industry which use substantial amounts of fossil fuels, such as transport, logistics and the production industry. These sectors’ energy needs are currently mainly supplied by fossil fuels, which need to be replaced by regenerative electricity or renewable fuels.
An alternative to fossil fuels are power-to-X fuels, which are synthesised from electrolysis-based hydrogen. Nowadays, the most important power-to-X fuel is hydrogen itself. Nevertheless, there are still considerable challenges to overcome in terms of transport and storage before hydrogen can be used as an energy source on a widespread basis. These include high space requirements for storage and/or other energetically disadvantageous conditions. Ammonia (NH3) could become an alternative solution that allows to store and transport the required hydrogen more easily.
Since ammonia can be liquefied at a moderate temperature of -33 degrees Celsius (compared to -253 degrees Celsius for hydrogen), its volumetric hydrogen content is significantly higher than for hydrogen compressed at 700 bars. Liquefied ammonia, in comparison to hydrogen, eases the transport of large volumes to where it is needed. As hydrogen produced from ammonia contains no carbon oxides or methane, it is also free of greenhouse gases.
Environmental impact and sustainable approaches
The ammonia cracking process has a significant environmental impact due to its energy consumption and associated emissions. Nonetheless, there are also sustainable approaches that can mitigate these negative effects. Below, environmental considerations are addressed and possible sustainable solutions are discussed:
Environmental Issues:
- Energy Consumption: Energy consumption in the ammonia cracking process is a critical issue and can be quite significant due to the elevated temperatures required to decompose ammonia into its components, nitrogen and hydrogen. The energy consumption is due to many reasons.
-
- High temperature: To conduct the decomposition of ammonia into nitrogen and hydrogen, high temperatures are required. These temperatures typically range from 800 to 1000 degrees Celsius, which involves substantial energy consumption to heat the reactants and keep the reaction at the appropriate temperature.
- Endothermic process: Ammonia cracking is an endothermic reaction, which means that it absorbs energy in the form of heat instead of releasing it. This feature increases the energy consumption even further, as a constant heat source is needed to keep the reaction ongoing.
- Pressure: In addition to temperature, pressure can also affect energy consumption. Increasing the pressure can favour the formation of desired products, but this requires an additional investment of energy to keep a high-pressure reaction ongoing.
- Catalysts and efficiency: The choice of catalysts used in the process can influence energy efficiency. Some catalysts can reduce the temperature required for the reaction, thus leading to a reduction in energy consumption.
-
- CO2 emissions: When natural gas or oil are used as an energy source, the ammonia cracking process can generate carbon dioxide (CO2) emissions, contributing to climate change. CO2 is an undesirable by-product of hydrogen and nitrogen production.
- Waste Management: Proper management of by-products and chemical waste generated during the ammonia cracking process is essential to avoid both air and water pollution.
- Safety: The handling of ammonia and high temperatures can be hazardous. Safety of workers and prevention of leaks are critical issues in the ammonia cracking industry.
Sustainable Approaches:
- Clean Energy: Using renewable energy sources, such as solar or wind power, to feed the ammonia cracking process can significantly reduce carbon emissions. This is known as “green hydrogen production”, where the hydrogen is produced by electrolysis of water using renewable electricity.
- Carbon Capture and Storage (CCS): Carbon capture and storage is a technology that allows CO2 emissions from the ammonia cracking process to be captured and safely stored, preventing them from being released into the atmosphere.
- Process Optimisation: Improving the efficiency of ammonia cracking processes can reduce the amount of energy required and therefore emissions. This includes the choice of efficient catalysts and precise control of reaction conditions.
- Circular Economy: Encouraging the reuse and recycling of by-products and chemical waste can reduce environmental impact and promote sustainability throughout the life cycle of this process.
- Heat and energy recovery: Waste heat recovery and process integration are key strategies to increase energy efficiency. Heat released during ammonia cracking can be captured and reused in other parts of the process or to heat feedstocks.
- Development of New Technologies: Ongoing research into innovative technologies, such as the production of hydrogen from biological sources or the development of more efficient catalysts, can lead to significant advances in the sustainability of ammonia cracking.
These strategies and technologies illustrate how innovation and a focus on sustainability can contribute to making ammonia cracking more efficient and eco-friendlier. As research progresses and innovative solutions are deployed, it is possible to achieve more sustainable hydrogen and nitrogen production with less dependence on non-renewable resources.
Conclusions
The ammonia cracking process is an essential part of several industries, supplying hydrogen and nitrogen, two key components in a wide variety of industrial applications. However, this process faces significant challenges in terms of energy consumption and environmental impact. To overcome these challenges, innovative strategies and technologies are being developed.
Transitioning to renewable energy sources, such as green hydrogen, and improving the efficiency of reactors and catalysts are key factors towards reducing energy consumption. Additionally, carbon capture and storage can help mitigate process-related CO2 emissions.
The responsible management of by-products and waste, as well as the embrace of emerging technologies such as advanced electrolysis, are also important steps in the pursuit of more sustainable hydrogen and nitrogen production.
Altogether, these innovations and sustainable approaches aim to make ammonia cracking more efficient and less damaging to the environment, contributing to a cleaner and more sustainable industrial future. Ongoing research and development in this field is essential to boost the evolution of the industry and meet global sustainability goals.
REFERENCES