Hydrogen storage and transport methods
“Hydrogen at €1.5/kg” is probably the most repeated slogan about hydrogen among politicians, manufacturers and companies over the last couple of years. The reason is clear: that is the price at which renewable hydrogen would be competitive compared to fossil fuels in most fields.
However, this comparison is certainly unfair, as once we dig deeper into the analysis it is far from reality. This is because these 1.5 €/kgH2 are just the production price of hydrogen, which is far from the actual cost of supplying it on time and form required by the client.
After all, the main challenge in the hydrogen industry is no longer how hydrogen is produced or at what price, but how it is stored, transported and delivered to the end-customer. That will be the key challenge for the upcoming years.
Storing and transporting hydrogen is not new. Hydrogen has been compressed or liquefied for industrial transport and storage for years. Moreover, some of the most famous hydrogen carriers, such as ammonia, methanol or liquid organic carriers (LOHC), have already been hydrogenating molecules for decades to produce these compounds for industrial purposes rather than for transporting and storing energy as hydrogen, which is precisely the purpose they will be used for over the next few years.
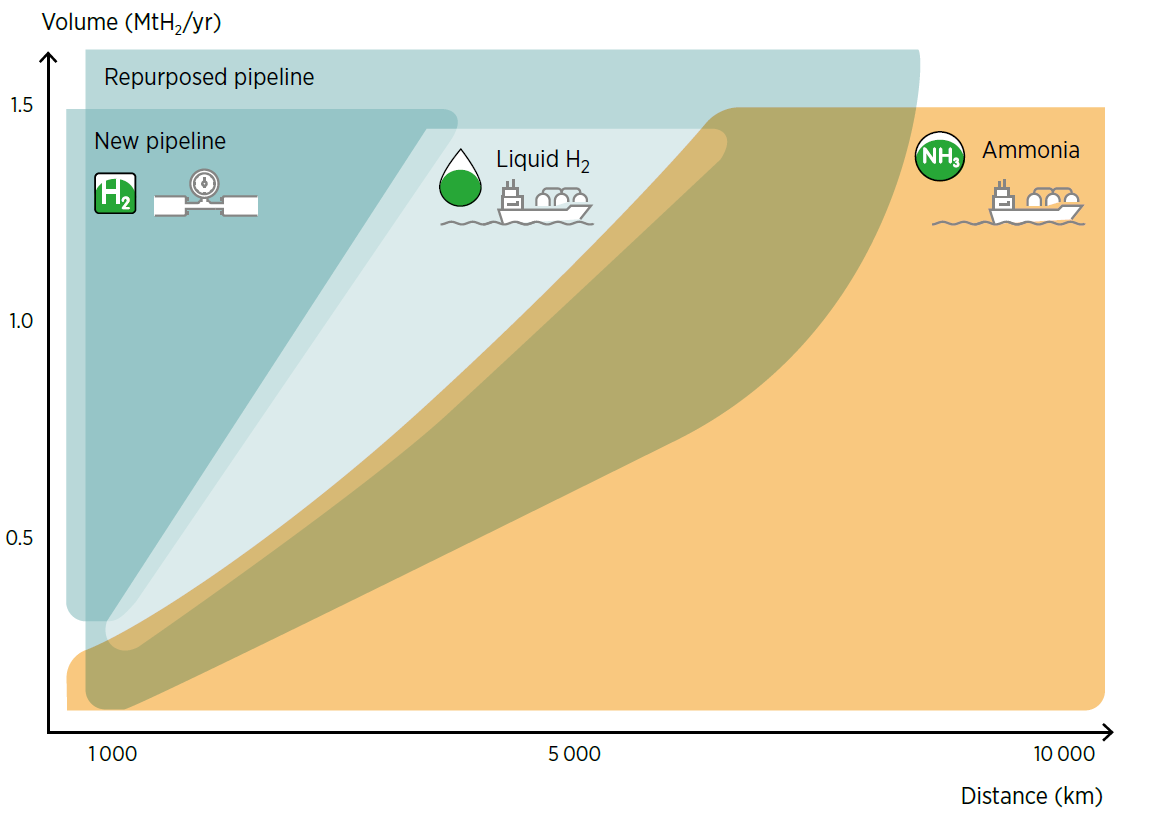
Figure 1. Transport procedures based on distance and volume according to IRENA
We are therefore not lacking in possibilities, but the optimal transition would require an understanding of the advantages and disadvantages of each alternative to enable the most efficient and least costly possible movement and delivery of hydrogen.
Let us explore the main methods we expect to witness in our energy systems within the next few years:
1.Compressed hydrogen
The most well-known and most widely used method so far. Hydrogen can be compressed and stored at different pressures (from 30 to 1,100 bar) in accordance with the end user or to the space requirements, by just using a compressor and storage tanks. It is important to know the end user at this point as to avoid wasting energy on unnecessary compressions. It is also important to optimise the number of tanks required, as the lower the pressure, the more tanks will be needed to store the same amount of hydrogen. The low density of hydrogen means that the first stage of compression (up to 30 bar) is very expensive in energy terms, so electrolysers that already supply hydrogen under pressure often offer advantages where hydrogen needs to be compressed.

Figure 2. PCS-related energy cost for hydrogen compression
Tube trailers are used to transport hydrogen in its compressed state. These tank trucks are already widely used and visible on roads. Most of these trucks are currently used to deliver hydrogen to smaller consumers and usually use pressures of 200 bar and metal cylinders. Over the next few years, it will be possible to transport hydrogen by road at pressures of up to 634 bar, versus the 500 bar maximum used today with composite tanks.
A further solution would be the use of pipelines for transporting the gas. Pipelines are well suited for both long and short distances, but they require large amounts of hydrogen to be cost-effective, and are therefore not expected to be widely implemented in these early years of hydrogen development.
2. Liquid hydrogen
When it comes to storage and transport, we must, without a doubt, increase the volumetric density of the component, in order to transport more hydrogen more effectively. So, what is the natural method after compression? Precisely, liquefaction of gas.
Hydrogen in its liquid state is much more dense than in its gaseous state (70 kg/m3), meaning that it can be stored in less space and transported in fewer trips. In addition, the storage tanks themselves are cheaper than compressed hydrogen would be. So far so good, yet with hydrogen… there is always a “but”! The main disadvantage here involves the large investments that are required to liquefy hydrogen and, above all, the high energy costs for cooling an element down to -253 °C, at which it changes to its liquid state. This energy cost is currently about 10-12 kWh/kgH2, meaning that approximately a third of the energy contained in hydrogen is consumed during this process. Optimism is high, and several companies and studies suggest a consumption as low as 6 kWh/kgH2, but only for large facilities of about 50 tonnes of hydrogen per day. However, this is still far away from the 2.19 kWh/kgH2 that is theoretically required to reach this temperature (it differs according to the inlet gas stream conditions).
There is one feature of this process that is particularly interesting, which complicates the hydrogen business in particular, namely the Joule-Thomson effect. By this effect, when gases expand under atmospheric conditions, they experiment a change in their temperature. Usually, gases cool down when they expand, but hydrogen, as usual, does not follow this effect, heating up when it expands. This always happens when the hydrogen is above -73 °C, which is known as the reversal temperature. Once below this temperature, the hydrogen cools down as it expands, and thus we reach -253 °C. Down to -73 °C, hydrogen is cooled by heat exchange with other fluids which do lower its temperature as it expands.
Liquid hydrogen has an assured future, both in intercontinental transport and in mobility (trucks, buses and planes). However, the scale requirements still make it a risky bet today. In my opinion, the first country to decide on doing it, will set a milestone in the field. Not by chance, those who understand these issues (Germany or the USA) are investing in research and development efforts for this method. There is already a boat (Suiso Frontier) on trial to transport liquid hydrogen. Its owners (Kawasaki heavy Industries) must be delighted by the results, since they have already applied for a licence to build an 11,200 tonne liquid hydrogen ship ( 130 times the capacity of the Suiso Frontier).
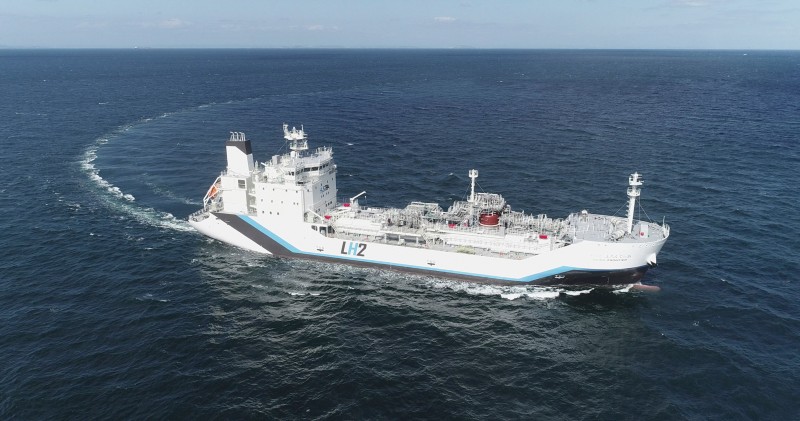
3. Ammonia
When it comes to intercontinental transport, ammonia is likely to be the favourite option. This is understandable since ammonia is an extremely energy-dense molecule that can hold more hydrogen per unit volume than liquid hydrogen itself, and which can be kept in a liquid state without significant effort, either by cooling to -33°C or by raising its pressure to 10 bar. Nowadays, this compound is produced by means of the Haber-Bosch process, which has been essential for the development of agriculture over the last 100 years and it is widely known.
Moreover, considering the large projects that are being planned globally, such as in Africa, Australia, Brazil, Chile, etc., the vast distances that separate these locations from the consumption sites, lead to the question of what type of transport method to use, with the great advantage that nitrogen is obtained from air, which means there will not be a shortage of feedstock anywhere on the planet.
It is also positive for ammonia that there is already an infrastructure and methodology to handle it, so energy systems would be more easily adjusted to it. The idea is to be able to use it as a carrier, which means that when the hydrogen arrives at the consumption site, is separated from the nitrogen so it can be used in its pure form. Nonetheless, it seems that ammonia could have enough demand (power generation, propelling ships, or already ongoing applications) to require the deployment of cracking units, which would imply additional investment and higher energy losses during the conversion process.
The main disadvantage of ammonia, as with other hydrogen carriers, is the high investment and energy costs involved during conversion and reconversion processes, which have a negative impact on the cost of the hydrogen provided. A successful integration with the surrounding equipment would allow the use of surplus heat in the Haber-Bosch process or the supply of external heat to the cracking unit, thus improving the efficiency of the hydrogen supply chain.
4. Liquid organic carriers
Liquid organic carriers are aromatic compounds that can be hydrogenated and dehydrogenated without decomposition and in a cyclic fashion. These compounds are widely used in industry and could acquire further value to the industry over the forthcoming years thanks to their role as sponges that absorb hydrogen and release it whenever and wherever requested. They are petroleum derivatives that are liquid and stable at atmospheric temperatures, both hydrogenated and dehydrogenated, and can hold hydrogen for a long period of time. Such carriers also have the advantage of being able to use the same infrastructure currently used for the transport of petroleum products, thereby granting them a second life within a sustainable economy. However, on the disadvantages, requiring hydrogenation and dehydrogenation processes involves investments and energy costs that lead to higher prices for the final hydrogen. Moreover, whenever this sort of solution is considered, it is essential to keep in mind closed planning chains, given the fact that the hydrogenated carrier must be returned dehydrogenated, to provide the hydrogen manufacturer a compound in which to store and transport the hydrogen molecule. Therefore, either the trucks or the ships would always be full of product and would have to do round trips, thereby also compromising the profitability of the transport.

Figure 3. Hydrogenation unit (Hydrogenious)
5. Methanol
This product will undoubtedly play one of the most important roles for the hydrogen sector in the future. Methanol is produced by combining CO2 and water through a water-gas shift reaction. Methanol or, for instance, synthetic methane – not included in this article as it is considered less interesting than its peers – is generated depending on the temperature and catalyst involved.
Methanol is already being used in industry nowadays, but also offers a wide range of possibilities within the energy sector for storing energy temporarily, generating electricity in large quantities, generating heat in industry (as a substitute of fuel oil) and even as a fuel used in transport, such as ships, but also for internal combustion vehicles, as there are already vehicles with 100% methanol in their tanks (especially in China).
Furthermore, methanol will be the base for many of the advanced fuels of the future, so the development of plants to produce synthetic methanol out of H2 seems to be highly justified. This only makes sense if we have a CO2 source available nearby.
The following graph shows the different energy, volumetric and gravimetric densities of hydrogen storage and transport methods:

Figure 4. Energy densities of different hydrogen storage and transport options
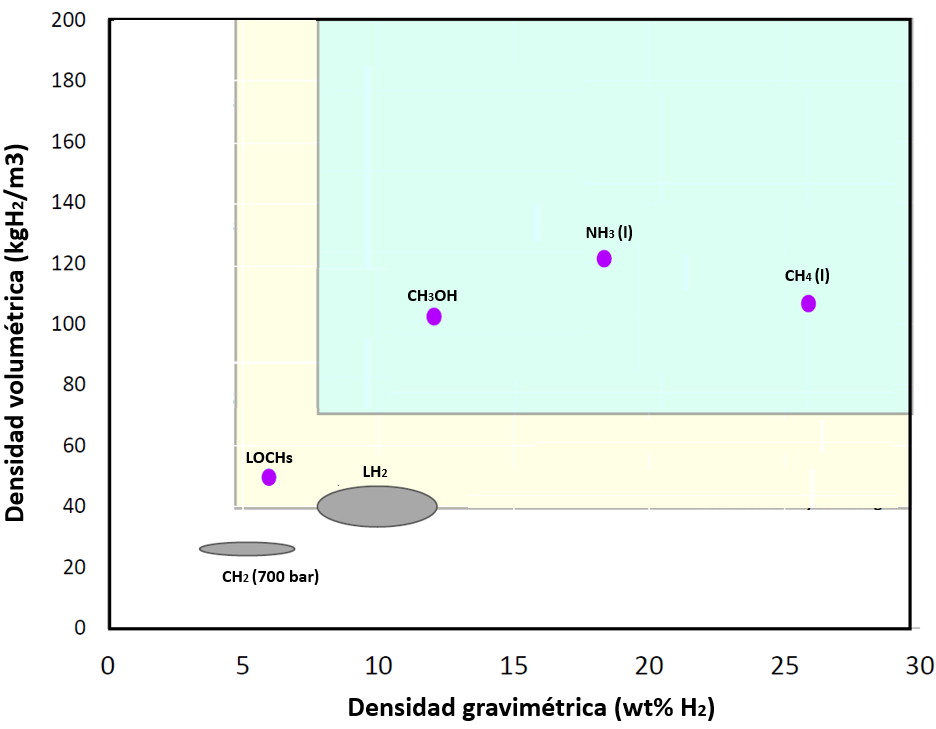
Figure 5. Volumetric and gravimetric densities of hydrogen storage and transport methods
We hope you have been interested in this brief overview of some of the different methods of storing and transporting hydrogen. Depending on the end use, as well as the availability of the resource at its origin, will determine which method is most suitable. At @SynerHy we develop studies aimed at making investment decisions regarding how to export or import renewable hydrogen. If you are interested in, please do not hesitate to contact us.